Written by Adeel Abbas
Key points
- Nucleophilic aromatic substitution is a chemical reaction where a nucleophile replaces a leaving group (such as a halide) on an aromatic ring.
- The reaction is activated by electron-withdrawing groups on the aromatic ring, and strong nucleophiles are required for the reaction to occur.
- The mechanism for nucleophilic aromatic substitution can be divided into two types: the addition-elimination mechanism and the benzyne mechanism (elimination-addition).
- The addition-elimination mechanism involves the formation of a negatively charged sigma complex, which is stabilized by resonance with electron-withdrawing groups on the aromatic ring. The chloride leaving group is then replaced by the nucleophile, and the product is deprotonated.
- The benzyne mechanism is used for unactivated halobenzenes and involves the abstraction of a proton by a strong base, leading to the formation of a carbanion intermediate. This intermediate can then react with the halogen to form the final product.
- Applications for nucleophilic aromatic substitution are more limited compared to electrophilic aromatic substitution.
In organic chemistry there’s a type of chemical reaction where a certain type of molecule called a nucleophile, can take the place of another molecule (called a halide) that is bonded to an aromatic ring (Benzene). This reaction is called nucleophilic aromatic substitution.
Think of it like a game of musical chairs, but with molecules instead of people and only certain types of molecules can play.”
Halide ions from aryl halides can be removed by nucleophiles, especially if the aryl halide has strong ortho or para electron-withdrawing groups. This type of reaction is known as a nucleophilic aromatic substitution because a nucleophile replaces a leaving group on an aromatic ring. The examples below demonstrate how 2,4-dinitrochlorobenzene can lose chloride when ammonia and hydroxide ions are present.
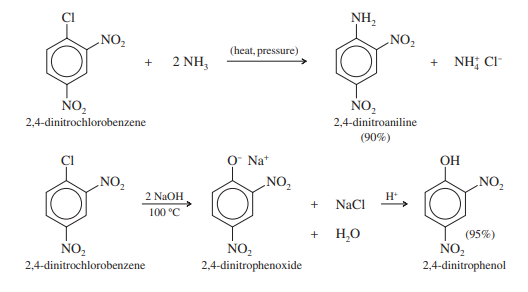
Applications for nucleophilic aromatic substitution are substantially more limited than those for electrophilic aromatic substitution. A potent nucleophile replaces a leaving group, such as a halide, in nucleophilic aromatic substitution. Since aryl halides are unable to acquire the proper geometry for backside displacement, the process cannot be the SN2 mechanism. The aromatic ring prevents the nucleophile from getting close to the carbon atom that is carrying the halogen.
The mechanism cannot be involved either. Strong nucleophiles are required for nucleophilic aromatic substitution, and the reaction rate is proportional to the concentration of the nucleophile. Thus, the nucleophile must be involved in the rate-limiting step.
The ring is activated for nucleophilic aromatic substitution by electron-withdrawing substituents (such as nitro groups), which may indicate that the ring is developing a negative charge in the transition state. In fact, the absence of at least one strong electron-withdrawing group makes nucleophilic aromatic replacements challenging. (In contrast to electrophilic aromatic substitution, where electron-withdrawing substituents cause the reaction to slow down or stop, this effect is the opposite).
Nucleophilic aromatic substitutions have been studied in detail. Either of two mechanisms may be involved, depending on the reactants. One mechanism is similar to the electrophilic aromatic substitution mechanism, except that nucleophiles and carbanions are involved rather than electrophiles and carbocations. The other mechanism involves “benzyne,” an interesting and unusual reactive intermediate.
The Addition–Elimination Mechanism
Table of Contents
Think about how sodium hydroxide and 2,4-dinitrochlorobenzene react.
A negatively charged sigma complex is created when the nucleophile hydroxide hits the carbon-containing chlorine. The ortho and para carbons of the ring and the electron-withdrawing nitro groups serve as delocalization sites for the negative charge. 2,4-Dinitrophenol is produced when the sigma complex loses its chloride; in this basic solution, it is deprotonated.
The addition–elimination mechanism requires strong electron-withdrawing groups to stabilize a negatively charged sigma complex.
Step 1: Attack by the nucleophile gives a resonance-stabilized sigma complex.

Step 2: Loss of the leaving group gives the product.
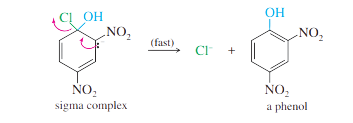
Step 3: This product (a phenol) is acidic, and is deprotonated by the base.
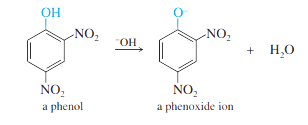
After the reaction is complete, acid would be added to deprotonate the phenoxide ion to give the phenol.
The resonance forms displayed in the mechanism box demonstrate how the stabilization of the intermediate (and the transition state leading to it) is aided by the nitro group ortho and para to the halogen. The development of the negatively charged sigma complex is rare in the absence of strong resonance-withdrawing groups in these places.
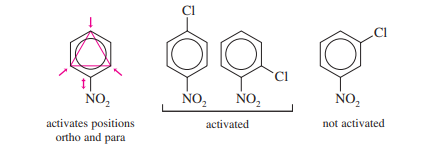
Fluoride ion is usually a poor leaving group because it is not very polarizable. Fluoride serves as the leaving group in the Sanger reagent (2,4-dinitrofluorobenzene), used in the determination of peptide structures.
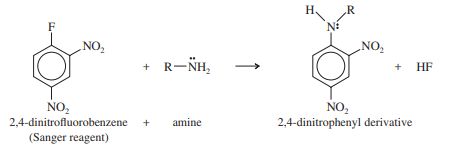
The Benzyne Mechanism: Elimination–Addition
Strong electron-withdrawing substituents on the aromatic ring are necessary for the addition-elimination mechanism of nucleophilic aromatic substitution. Unactivated halobenzenes do, however, react with strong bases in extreme circumstances. For instance, the “Dow process,” a commercial synthesis of phenol, calls for the 350 °C pressurized reactor treatment of chlorobenzene with sodium hydroxide and a little amount of water.
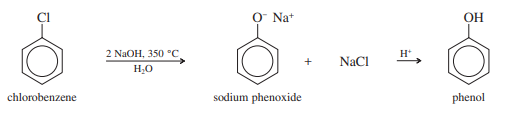
Similarly, chlorobenzene reacts with sodium amide ( an extremely strong base) to give aniline, This reaction does not require high temperatures, taking place in liquid ammonia at -33 °C.
Unactivated benzene derivatives undergo nucleophilic substitution by a different mechanism than the addition-elimination we observed with the nitro-substituted halobenzenes.
The interaction of sodium amide and p-bromotoluene offers a hint as to the mechanism. The products are a 50:50 mixture of m- and p-toluidine.
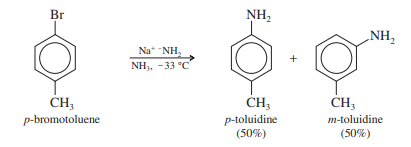
The elimination-addition mechanism, often known as the benzyne mechanism due to the uncommon intermediate, can be used to explain these two products. In the Dow process, sodium amide (or sodium hydroxide) interacts as a base, abstracting a proton. The end result is a carbanion, which has a negative charge and a pair of localized, non-bonding electrons in the orbital where the original bond was located.
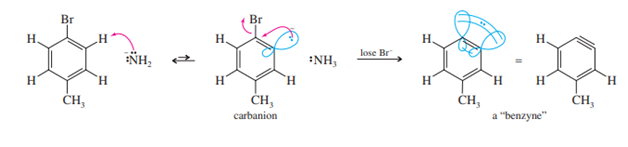
To change into a neutral species, the carbanion can release the bromide ion. An empty orbital is left behind as bromide departs with its bonding electrons. The filled orbital next to it overlaps with this orbital, adding to the bonding between the two carbon atoms. Since the two orbitals are pointed 60 degrees apart from one another, their overlap is not very useful. Due to the fact that a triple bond between these two carbon atoms may be used to represent this reactive intermediate, it is known as benzyne.
Triple bonds are typically linear; nevertheless, this triple bond is extremely reactive and greatly strained.
Amide ion is a strong nucleophile, attacking at either end of the weak, reactive ben[1]zyne triple bond. Subsequent protonation gives toluidine. About half of the product results from an attack by the amide ion at the meta carbon, and about half from an attack at the para carbon.
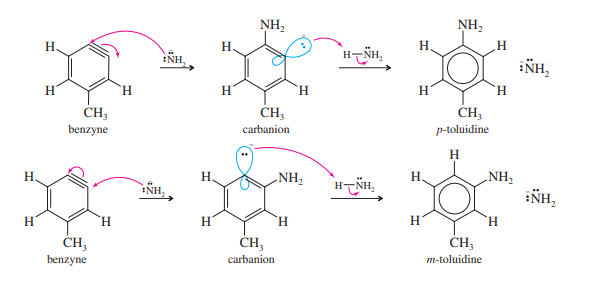
In conclusion, the benzyne mechanism functions under forcing conditions with a strong base when the halobenzene is inactive toward nucleophilic aromatic substitution. A reactive benzyne intermediate is created during a two-step elimination. The substituted product is produced by protonation after a nucleophilic attack.
Nucleophilic Aromatic Substitution (Benzene Mechanism)
The benzene mechanism (elimination–addition) is likely when the ring has no strong electron-withdrawing groups. It usually requires a powerful base or high temperatures.
Step 1: Deprotonation adjacent to the leaving group gives a carbanion.
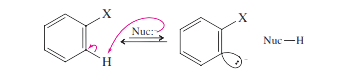
Step 2: The carbanion expels the leaving group to give a “benzyne” intermediate.
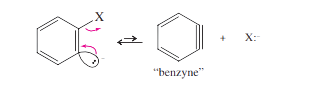
Step 3: The nucleophile attacks at either end of the reactive benzyne triple bond.
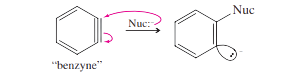
Step 4: Reprotination gives the product.
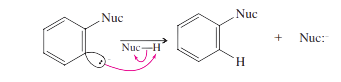
This is the general mechanism of Nucleophilic Aromatic Substitution.